Comparación de la propagación urbana en Helsinki, Finlandia
Una de las características más potentes de Wireless InSite® es la posibilidad de aplicar modelos y métodos de análisis de última generación a una amplia gama de problemas de propagación. Este ejemplo muestra el uso del modelo "Urban Canyon" para hacer predicciones de propagación urbana en un entorno microcelular en una sección de Helsinki, Finlandia. La simulación se compara con las mediciones y análisis del reciente artículo "Fast Two-Dimensional Diffraction Modeling for Site Specific Propagation Prediction in Urban Microcellular Environments" de W.Zhang, IEEE Transactions on Vehicular Technology, marzo de 2000 [1]. En la figura 1 se muestra el área de cobertura estudiada en [1], así como este ejemplo. Se encuentra cerca de una región de Helsinki conocida como "Plaza del Senado", en la posición E.
Para simular las características de propagación de esta parte de Helsinki, el usuario empieza por dibujar la huella de cada edificio utilizando el "editor de ciudades" de la interfaz de usuario de Wireless InSite (que se muestra en la figura 2) o importando un modelo de la ciudad en formato DXF. Utilizar el editor de ciudades es un procedimiento sencillo que consiste en hacer clic en los límites de cada edificio prestando atención a la escala y a los detalles del edificio. Para modelar la propagación urbana utilizando el modelo "Urban Canyon", no es necesario almacenar información sobre la altura de los edificios; todos los edificios se consideran mucho más altos que las ubicaciones del transmisor o del receptor, lo que permite una altura uniforme de los edificios de, por ejemplo, 50 metros. Una vez introducido el edificio, WI puede renderizar en 2D y 3D vistas ortográficas y en perspectiva de la ciudad. La figura 3 es una vista ortográfica en 3d de la sección de Helsinki que se va a modelar.
Figura 1: La zona de cobertura estudiada en [1], así como este ejemplo. Se encuentra cerca de una región de Helsinki conocida como "Plaza del Senado" en la posición E.
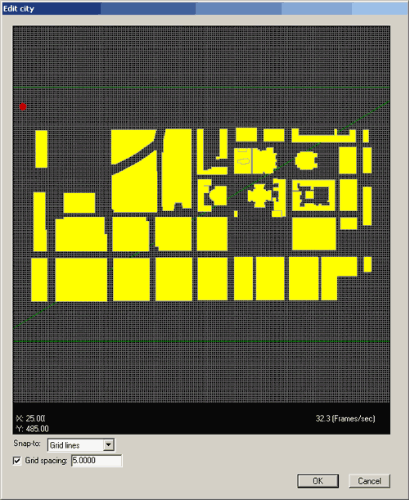
Figura 2: Editor de la ciudad que muestra los contornos 2D de los edificios de la zona considerada.
Figura 3: Vista en 3D del modelo del cañón urbano de Helsinki y su zona de estudio circundante.
Sin un conocimiento específico de los parámetros materiales de un edificio concreto, puede utilizarse un único material para toda la ciudad, como el ladrillo o el hormigón. Para este análisis se ha utilizado un material de construcción uniforme de hormigón con una constante dieléctrica de 5, como se hizo en [1]. Con información más detallada, se pueden asignar a cada edificio diferentes parámetros de material para cada una de sus caras, es decir, vidrio, acero, etc. Se cree que las zonas de la figura 1 que aparecen entrecruzadas son escaleras. Para iniciar este análisis se eliminaron, ya que no cumplen el requisito del modelo de cañón urbano de que las superficies verticales sean mucho más altas que las ubicaciones del transmisor o el receptor. No está claro en [1] cómo se modelaron estas regiones. Para determinar el efecto de las escaleras, se pueden realizar simulaciones con y sin esta característica.
Una vez definida la ciudad, se ajusta un terreno a la región en cuestión que también tiene parámetros de material. Para este análisis, se utilizó un semiespacio dieléctrico para el terreno con constante dieléctrica 25. Para añadir el terreno, haga clic con el botón derecho del ratón en la lista Características y, a continuación, Nuevo->Característica->Terreno. El terreno puede ajustarse automáticamente a las demás características (la ciudad en este caso) o puede especificarse por regiones concretas, como tierra y agua. Para cambiar los parámetros de material del terreno, utilice el cuadro de diálogo que se muestra en la figura 4.
Figura 4: Cuadro de diálogo de especificación de materiales.
Figura 5: Cuadro de propiedades de Rx en el proceso de definición de la ruta por la calle AOB
Una vez hecho esto, la lista de receptores se parecerá a la que se muestra en la Figura 6. Los transmisores se colocan de forma similar. La figura 7 muestra las rutas de receptores colocadas en la ciudad incluyendo el transmisor en el punto BS y el terreno. Una vez definidos los transmisores y receptores se puede asociar una antena a cada uno de ellos. Para este estudio se han añadido antenas similares a las utilizadas en el trabajo de Zhang [1]. Se utiliza una antena direccional de haz estrecho para los transmisores, y monopolos para cada punto receptor. Dado que el análisis se realiza en un plano 2D, también se puede utilizar una antena omnidireccional de polarización vertical para la recepción.
Figura 6: Ventana principal de Wireless Insite en la pestaña Receptores que muestra el conjunto completo de rutas de receptores de [1]. También se añadió una cuadrícula de 3528 receptores que cubre el área de estudio para producir un gráfico de superficie de la potencia recibida o pérdida de trayecto. Esto se muestra en la última línea.
Figura 7: Vista del proyecto que muestra las rutas del receptor y el transmisor.
Figura 8: Ventana principal con la pestaña Antena pulsada. En esta ventana se muestran ambas antenas para 900,5 y 1800 MHz.
A continuación se añade el Área de Estudio para encerrar completamente la ciudad. Inicialmente, el cálculo se realiza con el modelo de disparo de rayos Urban Canyon con 4 reflexiones, 2 difracciones y totalmente correlacionado. Las propiedades de los demás parámetros del cálculo están disponibles desde la ventana principal de Wireless InSite seleccionando la pestaña correspondiente y haciendo clic con el botón derecho del ratón sobre el elemento. Para la ejecución inicial, todos los receptores del proyecto están activos o en uso. Para realizar un estudio detallado de una calle o subdivisión en particular, los conjuntos de receptores que se encuentran fuera del área de especial interés pueden desactivarse para acortar el tiempo de ejecución. Ejecución de una simulación: El proyecto ya está listo para realizar los cálculos correspondientes a las ubicaciones de los transmisores y receptores que se muestran en la Figura 7. Después de guardar todos los archivos con un nombre adecuado, haga clic con el botón derecho del ratón en Proyecto>Ejecutar>Nuevo para iniciar el motor de cálculo. Una vez finalizado el cálculo, se pueden hacer comparaciones con los resultados encontrados en [1]. La pérdida de trayecto o la potencia recibida se pueden representar en función de la distancia o del número de receptores para cada una de las rutas de receptores definidas. En la Figura 9 se muestra la ruta a lo largo de la calle AOB y en la Figura 10 la ruta a lo largo de la calle KDC. El análisis puede realizarse a varias frecuencias en una sola ejecución. La predicción de la pérdida de trayecto a lo largo de LMN a 1,8 GHz se muestra en la Figura 11.
Figura 9: Gráfico de pérdida de trayecto a lo largo de la calle AOB que muestra los resultados del análisis y las mediciones de Zhang [1] en comparación con Wireless Insite.
Figura 10: Gráfico de pérdida de trayecto a lo largo de la calle KDC que muestra los resultados del análisis y las mediciones de Zhang [1] en comparación con Wireless Insite.
Figura 11: Comparación de pérdidas de trayecto a 1,8 GHz a lo largo de la calle LMN.
Los resultados de Wireless InSite no pueden reproducir los desvanecimientos a pequeña escala de las mediciones, ya que las ubicaciones y formas de los edificios no se conocen con gran precisión. Sin embargo, la concordancia es bastante buena. Si se dispusiera de datos más precisos sobre los edificios, se podrían colocar rutas de receptores cada 50 cm aproximadamente y guardar los campos eléctricos complejos correlacionados (una opción disponible haciendo clic en Avanzado en el cuadro de diálogo Propiedades del receptor).
Otra fuente de error tanto para los cálculos de Zhang como para Wireless Insite es que las mediciones se realizaron con cierto nivel de tráfico en las calles. Los efectos de los autobuses, camiones y coches no están presentes en los cálculos, pero tienen un efecto mensurable y dinámico en la propagación. Esto es probablemente más evidente cerca de las intersecciones M,O, y D donde la línea de visión (LOS) debería dominar si no hay tráfico. La subestimación de la pérdida de trayecto en estos lugares, tanto en el análisis de Zhang como en el de Wireless InSite, se debe en parte a la ausencia de tráfico de automóviles. Se pueden colocar algunas estructuras metálicas (simulando tráfico) en el trayecto para ver el efecto que tiene en la precisión. Esto se muestra en la figura 12. El análisis se vuelve a ejecutar con los nuevos obstáculos y se compara con el resultado sin tráfico en la figura 13. Es evidente que parte de la subestimación de la pérdida de trayectoria LOS podría deberse a la ausencia de tráfico.
Figura 12: Las estructuras rectangulares azules se añaden para simular el tráfico cerca de la intersección en AOB
Figura 13: Gráfico que muestra la diferencia en la predicción de la pérdida de trayectoria al considerar el efecto del tráfico de vehículos. El trazo rojo muestra la pérdida de trayectoria cerca del cruce sin tráfico y el trazo azul muestra el aumento de la pérdida de trayectoria debido a los pocos vehículos ficticios de la figura 12.
Para analizar el efecto de los pasos alrededor de DEF en la Figura 1 (crosshatch), El modelo se puede ejecutar con y sin subestructura que define los pasos. Pueden realizarse ejecuciones individuales para estudiar la zona de la calle BC. Las trayectorias individuales de los rayos pueden visualizarse para comprender mejor los mecanismos de propagación implicados en una zona determinada. En la figura 14 se muestra el análisis de la trayectoria de los rayos sin escalones y en la figura 15, con escalones.
Figura 14 . Estudio de los efectos de las escaleras alrededor de la Plaza del Senado. Se han eliminado las escaleras.
Figura 15 . La propagación por la calle BC con las escaleras presentes.
Figura 16 . Diagrama de superficie de la potencia recibida en Helsinki a 900 MHz.
Este fue un ejemplo de cómo Wireless Insite puede utilizarse para la predicción y planificación en entornos microcelulares urbanos y de la capacidad de igualar los métodos de análisis más avanzados para garantizar el éxito del diseño de primera pasada. Normalmente, para obtener resultados similares, el cálculo llevará unos minutos en una estación de trabajo Pentium 4 de 1 GHz.
Referencias
- "Fast Two-Dimensional Diffraction Modeling for Site Specific Propagation Prediction in Urban Microcellular Environments" por W. Zhang, IEEE Transactions on Vehicular Technology, marzo de 2000.